Direct Mass Measurements of Neutron-Rich Nuclides Below ^{100}Sn
After many years of R&D and tests, direct mass measurements of rare neutron deficient nuclides under challenging realistic conditions can be performed and shall demonstrate the overall performance of the FRS Ion Catcher. Projectile fragments in the range from Zr to Rh produced by projectile fragmentation of ^{124}Xe and ^{107}Ag are ideal to perform these measurements. They are refractive and can therefore be ideally produced and separated in-flight. Furthermore, depending on the ionization potentials of the various elements, the ions are expected to be thermalized in different charge states (+1 and +2). The controlled manipulation of charge states can thus be tested with these fragments. The mass measurements aim at N=Z and N=Z+1 nuclides in the element range from Y to Ag and will provide experimental mass values for many of these nuclides for the first time. These nuclides are of high interest because of their impact on the rp-process, isospin symmetry as well as pairing and the Wigner energy, the region of nuclear deformation in the vicinity of ^{80}Zr and the high-spin isomer in ^{94}Ag.
W. R. Plaß et al. Hyperfine Interactions 240:111 (2019)Direct Mass Measurements of Neutron-Rich Nuclides Below ^{208}Pb
The region south of ^{208}Pb nuclei along the N=126 line has been identified as a region of key interest for nuclear structure studies and nuclear astrophysics applications. This experiment aims to extend the frontiers of known isotopes and to obtain nuclear structure data on gross properties. The main goal of the experiment is to produce and identify new neutron-rich isotopes in the element range between terbium and rhenium and to measure their production cross sections, masses and half-lives. At the final focus of the FRS, the FRS Ion Catcher and detectors of the DESPEC collaboration (AIDA, FATIMA) will be used. It is the combined use of these novel instruments, which allows for these pioneering studies and the rich nuclear data harvest in short time.
W. R. Plaß et al. Hyperfine Interactions 240:111 (2019)
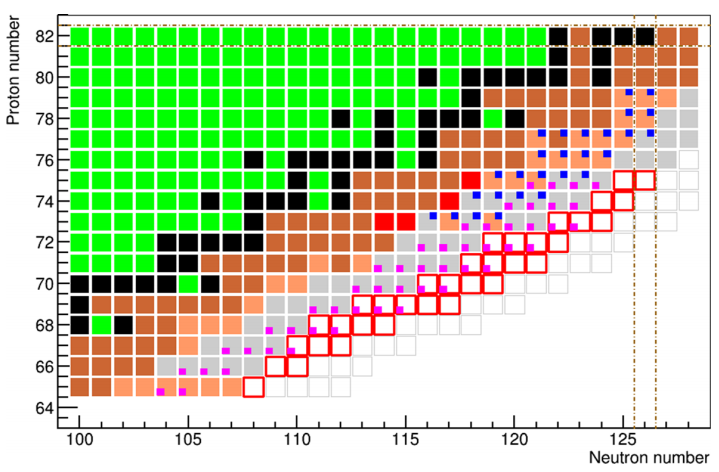
Reaction Studies With Multi-Nucleon Transfer (MNT)
Experiments with radioactive ion beams at Coulomb-barrier energies represent a new field for reaction studies which will contribute to a better understanding of multi-nucleon transfer, deep inelastic, fusion-fission and complete fusion reactions. One of the main goals of these experiments is to produce new neutron-rich heavy and super heavy isotopes, not reachable in fission or fragmentation reactions. Slowed-down rare ion beams of all chemical elements, including refractory elements, independent of their chemical properties, are available at the FRS and Super-FRS. Their optimum use requires a new philosophy for experiments and instrumentation. For instance multi-nucleon transfer (MNT) reactions inside the cryogenic stopping cell (CSC) of the FRS Ion Catcher is a unique and novel method that is very sensitive, clean, universal and has a high angular acceptance for the reaction products. It is planned to mount targets (e.g., Dy, Pt, U) on a wheel which will be installed in the stopping volume of the CSC. The identification and quantification of the reaction products will be performed by a high resolution and broadband mass spectrometer, which allows to identify the reaction products completely by their mass-to-charge ratio. This will pave the way for broad range measurements with primary and secondary beams. The concept is based on the uniqueness of the beams and experimental setups of GSI/FAIR and their combination.
In a first experiment we will perform MNT reaction studies with a 500 MeV/u ^{238}U primary beam slowed-down to Coulomb barrier energies impinging on different targets. We expect to measure more than 150 cross sections and 15 new masses of neutron-rich isotopes. The cross-sections will be used to benchmark reaction models. About the new masses, the proposed nuclei are important for the rare earth abundance peak in the r-process as well as for the understanding the properties of fissioning nuclei involved in the r-process. This is the first step towards future broad-range reaction studies with secondary beams at the (LEB of the Super-) FRS.
W. R. Plaß et al. Hyperfine Interactions 240:111 (2019)
Measuring β-Delayed Neutron Emission Probabilities
β-delayed single- or multi-neutron emission is possible when a mother nucleus β-decay Q-value ( Q_{ b} ) is larger than the daughter nucleus one- or x-neutron separation energy (S_{ 1n }or S_{xn}). The probability for β-delayed neutron emission ( P_{xn} for x emitted neutrons) increases with neutron number per isotope. Measured P_{ xn } values provide important input to the astrophysical r-process, nuclear structure models, and control of nuclear reactors. { P }_{ xn } data is scarce, especially for multi-neutron emission. For present and near-future accessible isotopes, less than 10% of multi-neutron emission candidates have been measured. Moreover, in the fission-product region, the most relevant for the r-process and nuclear reactors, there is published { P }_{ 2n} data for only four nuclides. Therefore, there are several world-wide campaigns to bridge this data gap, mostly by counting β-delayed emission n-β coincidence events. In this method, efficiency decreases with x (higher number of coincident neutrons) and β and n detector efficiencies depend on prior energy spectra measurements or models. In addition, the measurement includes neutron background from the beam and cosmic rays. Other proposed methods count the recoil nuclides via gamma spectra, time-of-flight, or mass measurements, in a series of stopping cells and MR-TOF-MSs (RIKEN), or in the ESR (GSI). We propose a complementary and independent method for measuring P_{xn} at the FRS Ion Catcher, which circumvents the drawbacks of existing methods. Obtain separated isotopes with the FRS, contain them in the Cryogenic Stopping Cell (CSC) for periods long enough for decay, and identify and count the precursor and recoil isotopes with the Multiple-Reflection Time-of-Flight Mass-Spectrometer (MR-TOF-MS). For { P }_{ xn} extraction, the masses of the precursor and all recoils are measured simultaneously at the MR-TOF-MS with accuracy that enables their unambiguous identification. Ratios between the recoil isotopes infer { P }_{ xn} for all x (x=0 is regular β-decay) . The method has the same efficiency for all x since the spectrometer has a broad mass range, is independent of nuclear models, has practically no background and is sensitive to isomers. First planned measurements are of { P }_{ xn} of ^{ 135-137 }{ Sb } and ^{142}I , the heaviest and highest Z β_{2n} precursor measured yet, setting the stage for a survey of -delayed neutron emission, including the N∼126 region.
Fission Isomers
The ‘island’ of fission isomers identified in the actinide region (Z = 92 - 97, N = 141- 151) originates from multi-humped fission barriers, which can be described as the result of superimposing microscopic shell corrections to the macroscopic liquid drop barrier. For the first time, populating fission isomers by using the fragmentation of 1 GeV/u ^{238}U projectiles was tried rather than light particle induced reactions so far in use. Projectile fragmentation gives access to isotopes that are hard or impossible to reach by light particle reactions. In-flight separation with the fragment separator FRS allows studying fission isomers with half-lives as short as 100 ns. Most importantly, it provides beams with high purity and enables an event-by-event identification. Different detection methods, such as decay and mass spectrometry, have been used in the experiment to search for fission isomers within a half-live from 100 ns to 50 ms. The experiment to study fission isomers with the FRS at GSI has been performed within the framework of FAIR Phase-0.
J. Zhao, Proceedings of FAIR next generation scientists - 7th Edition Workshop (FAIRness2022)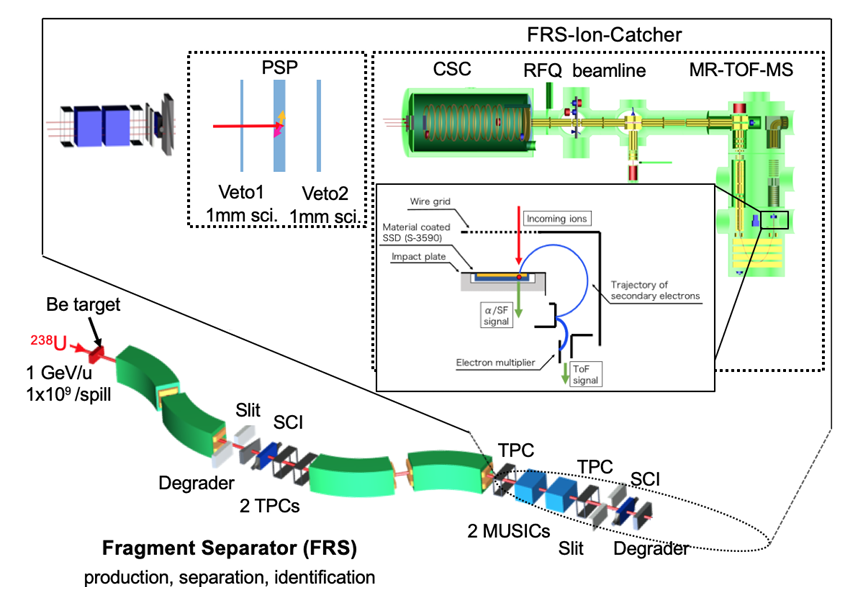